Dr. Christoph Förste
Head: Dr. Christoph Förste
Gravimetry has a long tradition on the Telegraphenberg hill in Potsdam, where the former Geodetic Institute started to carry out precise gravimetric pendulum measurements more than 100 years ago. Later on, spring gravimeters were used. Nowadays in our Section 1.2 at GFZ we apply two modern gravimetric techniques: Superconducting and Airborne Gravimetry.
According to Newton’s law the acceleration of free fall of a dropped body is proportional to its mass. That means the acceleration of free fall and the gravitational attractive force are equivalent. Therefore in Geodesy and Geophysics the acceleration of free fall is used as a measure for the gravity field.
Terrestrial gravity observations comprise the measurement of the vertical component of the acceleration of free fall g (g = Gravity) on the Earth's surface to determine spatial as well as temporal gravity variations. In this context one has to take into account that Gravity on the Earth’s surface is a superposition of the gravitational attraction of masses (Newton’s law) and the significantly smaller but outwards directed centrifugal acceleration due to the rotation of the Earth. Furthermore, mass density inhomogeneities as well as temporal mass displacements cause spatial and temporal variations of the gravitational attraction on the Earth’s surface which can be detected with gravity meters. In this context, spatial gravity observations can be used to derive information about geological structures such as fault zones, salt domes and volcanic structures or to explore ore deposits.
The largest signals of gravity variations are caused by the periodic changes of the relative positions between the Earth, the Moon and the Sun and the thereby induced deformations of the Earth's body (for instance Ocean and Earth tides and Ocean tide induced loading effects).
Examples of gravity variations on the Earth’s surface are (expressed as acceleration of free fall):
- The gravity difference between pole and equator due to Earth’s oblateness (the poles are closer to the Earth’s centre of mass and the centrifugal acceleration due the Earth’s rotation is zero at the poles and maximum at the equator):
Δg ~ 5•10-2 m/s² - Gravity differences between deep sea and highest mountains: Δg ~ 5•10-2 m/s²
- Earth tides: up to Δg ~ 3•10-6 m/s²
- Gravity changes by mass redistribution in the atmosphere: up to Δg ~ 2•10-7 m/s²
- Gravity changes by long-term terrestrial mass displacements: in the order of Δg ~10-7 m/s2
- Increase of Gravity due to 1 m groundwater rise: Δg ~ 4•10-8 m/s2
Superconducting Gravimetry
Temporal gravity variations can be recorded by using absolute and relative gravimeters. The most accurate and stabile relative gravimeters are Superconducting Gravimeters (SG). These instruments belong to the family of relative spring-mass gravimeters. In contrast to classical spring-mass gravimeters the SG has no spring. It’s replaced by a “virtual spring design” where a liquium helium cooled diamagnetic superconducting sphere is levitating in the super-stable magnetic field of a superconducting electromagnet.
As all gravity sensors a Superconducting Gravity meter is an integrating sensor measuring the sum of all gravity variations in its near and far environments. Therefore, the SG recordings include gravity effects of different sources. Due to the equivalence of gravitational and inertial mass (i.e. Einstein’s equivalence principle) the sensor inside a gravimeter (a test mass) is sensitive to temporal variations of Gravitational forces (Newtonian attraction) caused by redistribution and density variations of all surrounding masses as well as Inertial forces caused by accelerations, i.e. the second time derivative of the vertical position of the gravimeter site.
For the separation of the different gravity constituents sophisticated modelling and analysing techniques together with additional data (e.g. meteorological and hydrological data) are necessary. Research topics in this context are among others:
- Gravity changes induced by mass redistribution in the atmosphere and hydrosphere
- Comparison and Interpretation of satellite derived gravity variations
- Evaluation of ocean tide models and Hydrological models
- Inner core translation (Slichter Triplet)
- Core resonance in the tidal band (Nearly Diurnal Free Wobble)
- Polar motion and Free oscillations of the Earth
Presently about 30 superconducting gravimeters are worldwide operated within the IAG service International Geodynamics and Earth Tide Service (IGETS). “GFZ hosts the IGETS data base and contributes with the data of its own two superconducting gravimeters from the geodynamic observatories SAGOS (South African Geodynamic Observatory Sutherland) and ZUGOG (Zugspitze Geodynamic Observatory Germany).
South African Geodynamic Observatory Sutherland (SAGOS)
The South African Geodynamic Observatory Sutherland (SAGOS) of GFZ was established during the years 1998 – 2000 based on an Agreement on Cooperative Activities between the National Research Foundation (NRF) and GFZ, signed in August 1998. SAGOS is located at the site of the South African Astronomical Observatory (SAAO). The operation and maintenance of the SAGOS instrumentation is jointly done by staff of SAAO and GFZ.
The SAGOS observatory is located about 350 km north-east of Cape Town (longitude: 20.81 E, latitude: 32.38 S, altitude: 1755 m). The shortest distance to the South Atlantic coastline is about 200 kilometres. The area is located in a tectonically quiet zone far away from the African rift. Geologically, the setting is a huge dolerite plateau with a several kilometres thick layer of dolerite. This bedrock allows a good coupling of the Superconducting gravimeter (SG) pillars to the ground. The environment is a remote area with no industry and low seismicity. The climate at this place is determined by the border between summer and winter rainfall zones so that temperature fluctuations are not too rough. The observatory is also built into the ground to protect it against environmental effects like strong winds and temperature changes. All rooms are thermally insulated. An air condition system controls the temperature inside the measurement chamber, which is equipped with three concrete pillars embedded into the dolerite bedrock. Two of the pillars are constructed for Superconducting gravimeters or other geophysical instruments. The third pillar is dedicated for absolute gravimeters for the calibration of the Superconducting gravimeters. In the vicinity of the observatory four further pillars were set up for various other geodetic antennas and instrumentation.
SAGOS is a high precision geodynamic observatory comprising space techniques and ground instruments. Presently the observatory is equipped with
- One Superconducting Gravimeter (OSG D037), which is integrated in the IAG service International Geodynamics and Earth Tide Service IGETS. A second superconducting gravimeter (OSG 052) has been recording data between 2008 and 2017, before it was deinstalled in September 2017 for the operation at Zugspitze (ZUGOG). The data from these SGs are available for registered users from the IGETS data base hosted by GFZ. When using this dataset please cite it as follows
Förste, C., Voigt, C., Abe, M., Kroner, C., Neumeyer, J., Pflug, H., Fourie, P. (2016) Superconducting Gravimeter Data from Sutherland – Level 1. GFZ Data Services. http://doi.org/10.5880/igets.su.l1.001
SGs allow a stationary, continuous high-resolution monitoring of temporal changes of the Earth's gravity field. Main objectives are studying mass transport in the earth's system related to atmosphere, hydrosphere and analysing global geodynamic gravity processes such as polar motion, core modes, Earth & ocean tides and non-tidal loading effects.
- A local network of hydrological sensors (soil moisture, groundwater level, meteorology) in cooperation with Section 5.4 which are essentially needed for the interpretation of the SG measurements
- One permanent GNSS station (station ID: sutm) as a core station of the International GNSS Service (IGS). The data of this station are worldwide used within the geodetic science community for the determination of global and regional reference systems, for the investigation of crustal dynamics, for satellite orbit determination and others.
- One Triaxial Magnetometer, mainly used for investigations of the magnetic oscillation. Additionally, it is used as ground truth for satellite observations. The magnetometer has been installed in co-operation with the Hermanus Geomagnetic Observatory which permanently uses its data for instance in the international PLASMON project (coordinated by the Eötvös Loránd University, Hungary)
ZUGOG
The Zugspitze Geodynamic Observatory (ZUGOG) was established in September 2018 on top of mountain Zugspitze in the European Alps, with the dual targets of validation and calibration of GRACE-FO and supporting research into alpine mountain building processes and climate-relevant hydrological studies connected to the Environmental Research Station Schneefernerhaus. This second geodynamic observatory of GFZ includes the observatory superconducting gravimeter OSG 052, which observed previously in Sutherland, a permanent GNSS station and a local hydrological and meteorological sensor network. The superconducting gravimeter data are available for registered users from the IGETS data base hosted by GFZ. When using this dataset please cite it as follows
Voigt, C., Pflug, H., Foerste, C., Flechtner, F., Rehm, T. (2019) Superconducting Gravimeter Data from Zugspitze – Level 1. GFZ Data Services. http://doi.org/10.5880/igets.zu.l1.001
ZUGOG related publications
Timmen, L., Gerlach, C., Rehm, T., Völksen, C., Voigt, C. (2021): Geodetic-Gravimetric Monitoring of Mountain Uplift and Hydrological Variations at Zugspitze and Wank Mountains (Bavarian Alps, Germany). - Remote Sensing, 13, 5, 918.
https://doi.org/10.3390/rs13050918
Voigt, C., Schulz, K., Koch, F., Wetzel, K.-F., Timmen, L., Rehm, T., Pflug, H., Stolarczuk, N., Förste, C., Flechtner, F. (2021): Technical note: Introduction of a superconducting gravimeter as novel hydrological sensor for the Alpine research catchment Zugspitze. - Hydrology and Earth System Sciences, 25, 9, 5047-5064.
https://doi.org/10.5194/hess-25-5047-2021
HELGOG
The Helgoland Gravimetric Observatory Germany (HELGOG) was established in March 2020 in the basement of the Biologische Anstalt Helgoland (BAH) of the Alfred Wegener Institute AWI, Helmholtz Centre for Polar and Marine Research. The main research focus is on the analysis of tidal and non-tidal ocean loading in the North Sea for the validation and calibration of the satellite mission GRACE Follow-On. This observatory includes the superconducting gravimeter iGrav 047. The superconducting gravimeter data are available for registered users from the IGETS data base hosted by GFZ. When using this dataset please cite it as follows
Voigt, C., Stolarczuk, N., Pflug, H., Förste, C., Flechtner, F., Peters, H., Fietz, M. (2020): Superconducting Gravimeter Data from Helgoland - Level 1.
https://doi.org/10.5880/igets.he.l1.001
HELGOG related publications
Voigt, C., Sulzbach, R., Timmen, L., Dobslaw, H., Weise, A., Deng, Z., Stolarczuk, N., Pflug, H., Peters, H., Fietz, M., Thomas, M., Förste, C., Flechtner, F. (2023 online): A superconducting gravimeter on the island of Heligoland for the high-accuracy determination of regional ocean tide loading signals of the North Sea. - Geophysical Journal International. https://doi.org/10.1093/gji/ggad147
Weise, A., Timmen, L., Deng, Z., Gabriel, G., Rothleitner, C., Schilling, M., Voigt, C. (2020): Observing Ocean Mass Variability with Spring Gravimeters – Storm Surge Induced Signals on the North Sea Island Helgoland. - AVN Allgemeine Vermessungsnachrichten, 127, 4, 163-173.
Airborne and Ship Gravimetry
Satellite-based gravity field determination can map the gravity field of the Earth in a very homogeneous way, however, with limited spatial resolution due to the altitude of the orbits. On the other hand, traditional terrestrial gravimetry on ground can measure the gravity with high resolution, but its data are often inhomogeneous and such measurements can be limited by difficult environmental conditions like high mountains, glaciers, mush or jungle. Furthermore, traditional terrestrial gravimeters cannot be used on sea. Air- and shipborne gravimetry measurements can be used to fill data gaps of the traditional gravimetry on ground and the satellite techniques. Thanks to the development of GNSS, air- and shipborne gravimetry nowadays can operate routinely not only for research, but also for resource investigation etc. Regional gravity field models can be developed on the basis of this technique.
Specially designed, transportable spring gravimeters have been developed for use on aircraft and ships and are installed on gyro-stabilized platforms. These include GNSS receivers, whose measurements allow the necessary reduction of the non-gravitational, kinematic accelerations of the measurement platform. To measure the gravity field on ships or aircraft, special inertial navigation systems are increasingly being used, whose accelerometers have the necessary accuracy and drift stability. These devices, also known as strapdown gravimeters, are tightly fixed on the moving vehicle and work without a gyro-stabilized platform. Furthermore, there are now promising technological developments ongoing, that allow new quantum gravimeters to be used as mobile gravimeters on airplanes and ships.
For more than ten years, the GFZ has owned a mobile gravimeter of the Chekan-AM type, which was manufactured by the Russion company CSRI "Elektropribor"and received a new sensor unit in 2017. In the field of flight gravimetry, this device was used together with several JAVAD Delta G3T GNSS receivers as part of the GEOHALO mission. Later, intensive use in ship gravimetry took place over several years in the FAMOS project .
The GFZ has recently acquired a new iCORUS strapdown gravimeter from the manufacturer iMAR Navigation & Control. The iCORUS is currently used together with the Chekan gravimeter in ship gravimetry on the North Sea. The aim of this activity is determination of the geoid on the North Sea.
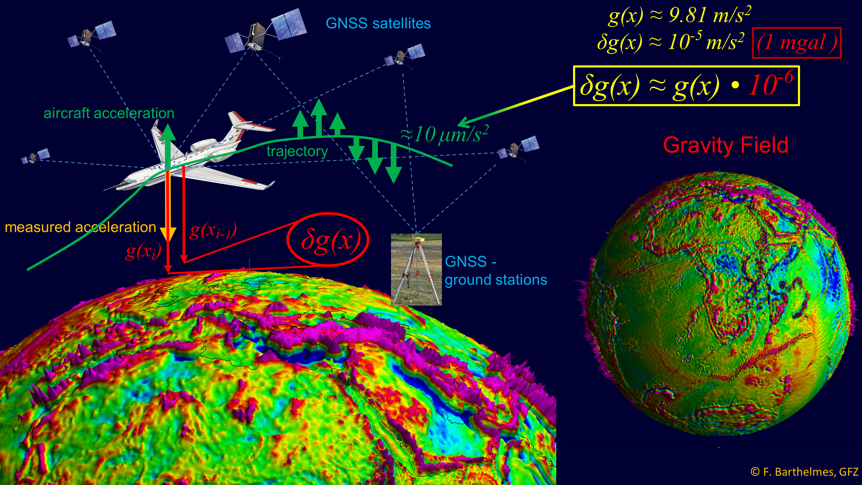
Literature
Boy, J.-P., Barriot, J.-P., Förste, C., Voigt, C., Wziontek, H. (2020 online): Achievements of the First 4 Years of the International Geodynamics and Earth Tide Service (IGETS) 2015–2019. - In: (International Association of Geodesy Symposia ), Berlin, Heidelberg : Springer-Verlag. https://doi.org/10.1007/1345_2020_94
Förste, C., Ince, E. S., Johann, F., Schwabe, J., Liebsch, G. (2020): Marine Gravimetry Activities on the Baltic Sea in the Framework of the EU Project FAMOS. - ZfV: Zeitschrift für Geodäsie, Geoinformation und Landmanagement, 145, 5, 287-294. https://doi.org/10.12902/zfv-0317-2020
Ince, E. S., Förste, C., Barthelmes, F., Pflug, H., Li, M., Kaminskis, J., Neumayer, K., Michalak, G. (2020): Gravity Measurements along Commercial Ferry Lines in the Baltic Sea and Their Use for Geodetic Purposes. - Marine Geodesy, 43, 6, 573-602. https://doi.org/10.1080/01490419.2020.1771486
Ince, E. S., Barthelmes, F., Reißland, S., Elger, K., Förste, C., Flechtner, F., Schuh, H. (2019): ICGEM – 15 years of successful collection and distribution of global gravitational models, associated services and future plans. - Earth System Science Data, 11, 647-674. https://doi.org/10.5194/essd-11-647-2019
Voigt, C., Schulz, K., Koch, F., Wetzel, K.-F., Timmen, L., Rehm, T., Pflug, H., Stolarczuk, N., Förste, C., Flechtner, F. (2021): Technical note: Introduction of a superconducting gravimeter as novel hydrological sensor for the Alpine research catchment Zugspitze. - Hydrology and Earth System Sciences, 25, 9, 5047-5064. https://doi.org/10.5194/hess-25-5047-2021